When an intense beam of charged particles or radiation propagates inside plasma, it can expel the plasma electrons away from the axis, forming wakefields akin to that seen in the ocean waves. These wakefields can sustain ultra-high electromagnetic fields capable of accelerating and focusing charged particles. These plasma-based wakefield accelerators can accelerate charged particles within a very short distance, giving rise to the name “tabletop accelerators”.

Figure 1. Synergistic implementations of plasma-based wakefield accelerators [1-2]. (a) Laser-Electron Plasma Beam Accelerator (LEPA), where the driving electron beam and laser pulse pump energy to each other, increasing the acceleration distance of the wakefield. (b-c) Electron density (b) and electron spatial distribution(c) in a laser wakefield accelerator where the electrons are gaining energy from both the plasma wakefield and the laser itself. Colors in (c) represent the electron kinetic energy in electron rest mass energy.
Our group uses computational and analytic tools to study a wide array of acceleration schemes, giving rise to new concepts. One such idea is the marriage between Direct Laser Acceleration (DLA) and PWFA. When an electron propagating in a focusing field can interact resonantly with a co-propagating laser field, the laser can directly gain energy from the laser’s transverse electric field. We have demonstrated the synergy of DLA with Laser-driven Wakefield Accelerator (LWFA) and beam-driven plasma wakefield accelerator (PWFA).
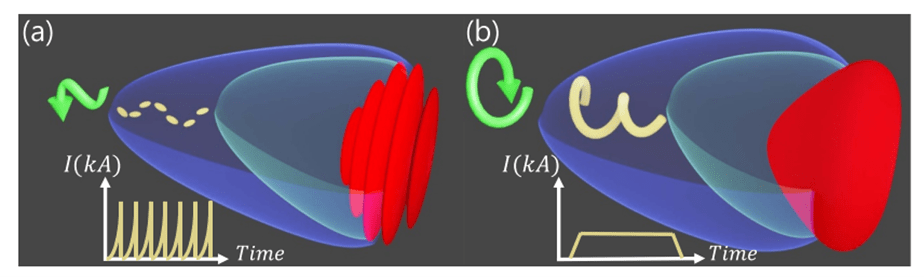
Figure 2. Schematic of electron injection into an Expanding Periodically undulating Bubble driven by (a) linearly polarized laser pulse and (b) circularly polarized laser pulse [3]. An expanding and transversely undulating bubble (early time: light blue, later time: dark blue, bubble back motion: green arrows) is formed by a laser with steepened front (intensity in red). According to laser polarization, periodically modulated or flat current beam (bunch density in yellow) can be generated. The graphs in the bottom with the white axes show injected current profiles (yellow lines).
Another key consideration for plasma-based accelerator is electron injection; the electron beams injected into the plasma wakefield determine the potential use or the subsequent acceleration dynamics. Our group has earlier demonstrated that the self-evolution of the laser pulse can inject electrons into the plasma bubble, using a semi-analytic Hamiltonian framework. We have recently extended this framework to describe the Carrier-Envelope-Phase (CEP) effect in laser wakefields. A laser pulse driven by a near single cycle pulse or steepened pulse executes periodic transverse undulations according to the evolution of laser phase at the peak intensity. We have found that these undulations can turn on/off electron injection from the background plasma, injecting complicated but regular spatio-temporally periodic electron bunches.

FIG. 3. (a) The 3D simulation domain in WAND-PIC[4]. The 2D transverse plane is partitioned into square subdomains. In the longitudinal direction, the adaptive step size is shown by the red ticks which are denser at the back of the bubble. (b) The multigrid approach shown is a hierarchy of grids in one subdomain with different mesh sizes.
Our group is also actively developing ways to efficiently simulate laser-plasma interactions. In particular, we have recently published a scalable, in-house simulation code using Quasistatic approximation. In this approximation, the faster plasma dynamics is uncoupled from the slower driver (laser pulse, relativistic driver beam) dynamics, enabling taking much longer time-steps, enabling computational speed-up by several orders of magnitude. Advanced algorithms enabling simulation of Direct Laser Acceleration or in-situ computation of X-ray radiation from the high-energy electrons are implemented.
References and Selected Publications:
[1] T. Wang, V. Khudik, and G. Shvets, Laser-pulse and electron-bunch plasma wakefield accelerator, Phys.Rev.Accel.Beams. 23, 111304 (2020)
[2] X. Zhang, V. Khudik, and G. Shvets, Synergistic Laser-Wakefield and Direct-Laser Acceleration in the Plasma-Bubble Regime, Phys. Rev. Lett. 114, 184801 (2015)
[3] J. Kim, T. Wang, V.Khudik, and G.Shvets, Polarization and phase control of electron injection and acceleration in the plasma by a self-steepening laser pulse, New. J. Phys, 25 033009(2023)
[4] T. Wang, V. Khudik, J. Kim, and G. Shvets, Accelerated three-dimensional quasistatic particle-in-cell code, Phys.Rev.Accel.Beams., 25, 104603 (2022)
[5] V. N. Khudik, A. Arefiev, X. Zhang and G. Shvets, Universal scalings for laser acceleration of electrons in ion channels, Phys. Plasmas 23, 103108 (2016)